Parts Group
Understanding human DNA function by engineering
Research overview
Approach
The following four statements describe our approach:
1) We get things done. We start projects with clearly defined goals, and publish both positive and negative results of the ones that pass the pilot stage. We deliver to our collaborators.
2) We work on important problems. We pick projects based on how much they impact our understanding of human cells, characterize the variation of gene function across individuals, or influence how others work.
3) We succeed as a team. We have a diverse mix of backgrounds and skillsets, complementing each other with our strenghts.
4) We are excited about science. We read broadly, discuss latest developments, and keep up to date both with the depth of our field, and the entire breadth of genomics.
Projects
Core team
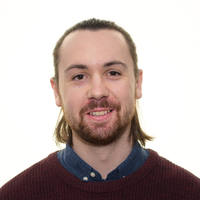
Dr Alistair Dunham
Postdoctoral Fellow
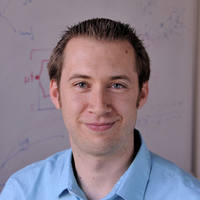
Mr Gareth Girling
Advanced Research Assistant
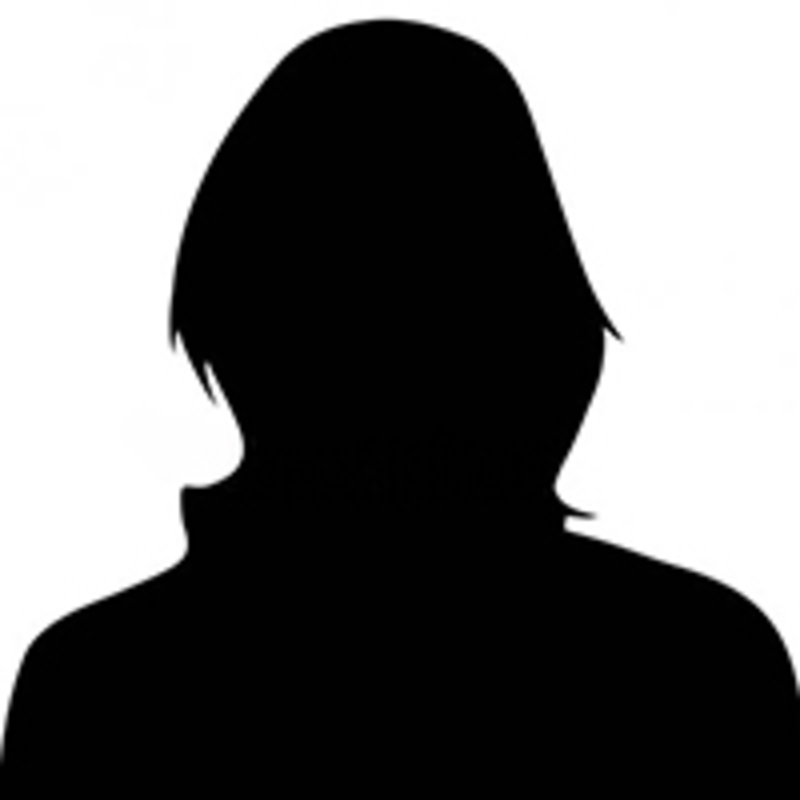
Elin Madli Peets
Advanced Research Assistant
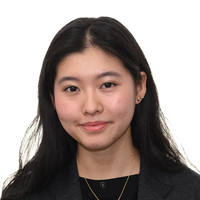
Isabelle Zane
PhD Student
Previous core team members
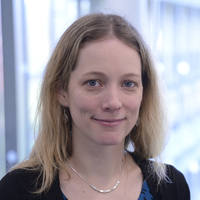
Dr Felicity Allen
Postdoctoral Fellow
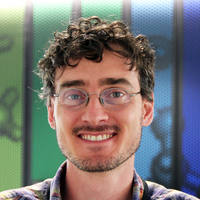
Luca Crepaldi
Staff Scientist
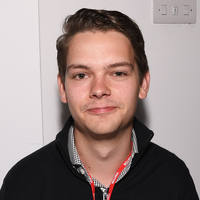
Jacob Hepkema
PhD Student
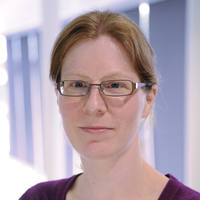
Dr Michelle McRae
Senior Research Assistant/Laboratory Manager
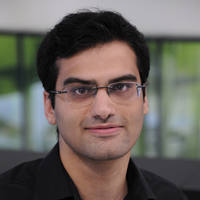
Danesh Moradigaravand
Senior Bioinformatician
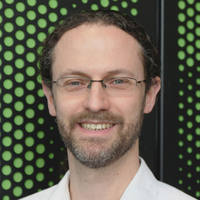
Dr Daniele Muraro
Senior Bioinformatician
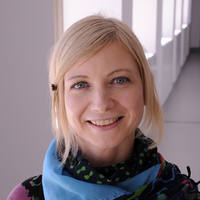
Kasia Tilgner
Visiting Scientist
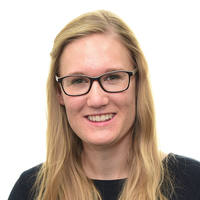
Juliane Weller
PhD Student
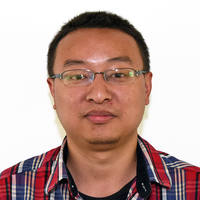
Dr Yan Zhou
Postdoctoral Fellow
Related groups
Programmes and Facilities
Partners
We work with the following groups
External
Cancer Dependency Map
The Cancer Dependency Map aims to find a targetable dependency in each cancer cell.